Database Menu
Single-Crystal Elasticity Links
COMPRES
Contribute to hklion/singleCrystal development by creating an account on GitHub. Monocrystalline silicon, more often called single-crystal silicon, in short mono c-Si or mono-Si, is the base material for silicon-based discrete components and integrated circuits used in virtually all modern electronic equipment. Mono-Si also serves as a photovoltaic, light-absorbing material in the manufacture of solar cells. It consists of silicon in which the crystal lattice of the.
This video is the first in a two-part series on how to grow a large, single crystal using alum. This video was produced by the University at Buffalo in partn. The single crystal materials were extremely resistant to oxygen loss below 100°C compared to the polycryst. The major drawback of the single crystal material is its slightly lower specific capacity compared to the polycryst. However, this may not be an issue for Li-ion cells designed for long lifetime applications. Application: Metal single crystals are required among others for basic research (surface physics, catalytic chemistry, investigation of material properties, etc.), for monochromators (for X-ray, neutrons, etc.) and electron sources (W-needles, LaB6-, CeB6-cathodes, etc.).
Introduction
This web site provides an introduction to the single-crystal second-order elastic moduli of minerals and related materials. The goal is to collect and systematize the large literature of single-crystal elasticity data reported on such materials over the past eight decades or so. A database is currently under development that contains data for more than 400 mineral compositions. In addition to the single-crystal elastic moduli, related quantities computed from the single-crystal elastic moduli (aggregate elastic properties, sound velocities, anisotropy factors, etc.) are compiled. A brief overview of the importance of mineral elasticity, statistics on the distribution of literature measurements, and some examples of overall trends in mineral elasticity are provided below, as well as links for many other resources. A preliminary version of the complete database is available upon request.
Thomas Duffy, duffy@princeton.edu
Renee Delamater-Droungas, reneed@princeton.edu
Department of Geosciences Princeton University
The Single-Crystal Elastic Moduli Are Fundamental Properties Of Minerals
Mechanical properties
- Stress-stain, compressibility
- Hardness, brittle-ductile behavior, damage, mechanical stability
Elastic wave propagation, anisotropy, interpretation of seismic data
Thermodynamic properties
- Equations of state
- Phase stability
- Debye temperature, specific heat, Gruneisen parameter
Interatomic interactions, lattice dynamics, nature of bonding
Anisotropic Elasticity
Single-crystal elastic moduli are a 4th rank tensor with 3-21 independent,
non-zero coefficients, depending on the crystal system.
Distribution of Single-Crystal Elasticity Data Among Mineral Groups and Crystal Systems
Oxides includes hydroxides; Carbonates includes nitrates, Sulfates includes molybdates and tungstates; carbides includes carbon, nitrides, phosphides.
Bulk Modulus vs Shear Modulus For Minerals
Aggregate bulk modulus vs shear modulus for minerals according to Nickel-Strunz class. Several trends are apparent. K/G typically ~1.5 up to K~200 GPa with K/G tending to decrease for higher values of K. Above K=100 GPa, oxides and silicates are dominant with other mineral classes generally have K<100 GPa. Oxides generally exhibit a higher K/G than silicates, reflecting a higher degree of ionic bonding. Selected minerals are labeled.
BACK TO TOP
Poisson’s Ratio vs Shear Modulus
Aggregate Poison’s ratio vs shear modulus, G, for the dataset. K/G values representative of different bonding types are shown. Maximum values of Poisson’s ratio for a given value of the shear modulus decreases with increasing G as shown by the solid red line.
BACK TO TOP
Elastic Anisotropy of Crust and Mantle Minerals
Elastic anisotropy of selected crust and mantle minerals at ambient conditions based on the universal anisotropy index, AU, which is determined from the Voigt and Reuss bounds of the bulk and shear moduli. The large difference in anisotropy between crustal and subduction zone minerals and those expected in the Earth’s mantle is clearly evident.
BACK TO TOP
Elasticity of SiO2 Polymorphs: Poisson’s Ratio
The aggregate Poisson’s ratio of SiO2 polymorphs is plotted as a function of density. Poisson’s ratio for SiO2 shows extreme variations as a function of pressure and temperature especially in the vicinity of martensitic phase transitions(a-b quartz, stishovite-CaCl2-type). Filled symbols are experimental data while solid lines with pluses show theoretical calculations. Both high pressure/ambient temperature and ambient pressure/high temperature data are shown. Below the horizontal dashed line at n=0 is the region of bulk auxetic behavior (negative Poisson’s ratio). The horizontal dashed line at 0.5 corresponds to the maximum Poisson’s ratio for a solid aggregate. The open circles in light grey show values of Poisson ratio values for other silicates at ambient conditions.
Acknowledgements:
This project is supported by COMPRES, the Consortium for Materials Properties Research in Earth Sciences under NSF Cooperative Agreement EAR 1606856.
Single crystal
In crystalline solids the atoms or molecules are stacked in a regular manner, forming a three-dimensional pattern which may be obtained by a three-dimensional repetition of a certain pattern unit called a unit cell. When the periodicity of the pattern extends throughout a certain piece of material, one speaks of a single crystal. A single crystal is formed by the growth of a crystal nucleus without secondary nucleation or impingement on other crystals. SeeCrystal structure, Crystal-
When grown from a melt, single crystals usually take the form of their container. Crystals grown from solution (gas, liquid, or solid) often have a well-defined form which reflects the symmetry of the unit cell. SeeCrystal growth
Ideally, single crystals are free from internal boundaries. They give rise to a characteristic x-ray diffraction pattern.
Many types of single crystal exhibit anisotropy, that is, a variation of some of their physical properties according to the direction along which they are measured. For example, the electrical resistivity of a randomly oriented aggregate of graphite crystallites is the same in all directions. This anisotropy exists both for structure-sensitive properties, which are strongly affected by crystal imperfections (such as cleavage and crystal growth rate), and for structure-insensitive properties, which are not affected by imperfections (such as elastic coefficients).
The structure-sensitive properties of crystals (for example, strength and diffusion coefficients) seem governed by internal defects, often on an atomic scale. SeeCrystal defects
Single Crystal
an individual crystal that has a continuous crystal lattice and is characterized by anisotropy of its properties. The external shape of a single crystal is determined by its atomic-crystalline structure and the conditions of crystallization. A single crystal often acquires well-defined natural faceting; under nonequilibrium conditions of crystallization, the faceting is weakly manifested. Single crystals of quartz, salt, Iceland spar, diamond, and topaz are examples of faceted natural single crystals. Polycrystals and polycrystalline aggregates, which consist of a set of small single crystals of various orientation, are distinguished from single crystals.
Single crystals are valuable as materials that have special physical propertes. For example, diamond and borazon are extremely hard, fluorite is transparent to a broad range of wavelengths, and quartz is piezoelectric. Single crystals are capable of changing their properties under the influence of external factors (light, mechanical strains, electric and magnetic fields, radiation, temperature, or pressure); therefore, products and components made from them are used as various types of transformers in radio electronics, quantum electronics, acoustics, and computer technology. Originally, natural single crystals were used in engineering, but supplies are limited and the quality is not always sufficiently high. At the same time, many valuable properties have been found only in synthetic crystals. Therefore, the necessity of growing crystals artificially has arisen. The parent substance for growth of single crystals may be a solid (particularly a powder), a liquid (melt or solution), or a gas.

Methods of growing single crystals from a melt include the Stockbarger, Czochralski, and Verneuil methods and zone melting. In the Stockbarger method a crucible containing a melt is moved vertically through a furnace at a rate of 1–20 mm/hr (Figure 1). The temperature in the plane of the diaphragm is kept equal to the crystallization temperature of the substance. Since the crucible has a conical bottom, when it is slowly lowered the melt in the cone is at a temperature below the crystallization temperature, and very small crystals form. Because of geometric selection, only one crystal survives. The selection is related primarily to the anisotropy of the rates of growth of the single crystal’s facets. This method is used widely in the industrial production of large single crystals of fluorite, lithium fluoride, cadmium sulfide, and other chemicals.

In the Czochralski method the single crystal is slowly pulled from the melt (Figure 2) at a rate of 1–20 mm/hr. This method makes possible the production of single crystals of specific crystallographic orientation. The Czochralski method is used in growing single crystals of yttrium-aluminum garnet, lithium niobate, and semiconductors. Using this method, Stepanov devised another method of growing crystals with a cross section of prescribed form.
The Verneuil method uses no crucible. The substance, in the form of a powder (particle size, 2–100 microns [LI]), is fed from a bin (Figure 3) through an oxyhydrogen flame to the upper, molten face of a seed single crystal, which is slowly lowered by a mechanism. The Verneuil method is the principal industrial method of producing refractory single crystals, such as ruby, spinel, and rutile.
In the zone melting method, a melting zone of extremely limited width is created, and the single crystal is produced by sequential melting of an entire ingot. The zone melting method is widely used in the production of semiconductor single crystals (W. G. Pfann, 1927) and such refractory single crystals as molybdenum and tungsten.
There are three methods of growing crystals from solution: the low-temperature method (using water, alcohol, and acids as solvents), the high-temperature method (using molten salts), and the hydrothermal method. A low-temperature crystallizer is a vessel containing a solution in which supersaturation, which is necessary for growing crystals, is created by means of slow lowering of temperature or, less frequently, by evaporation of the
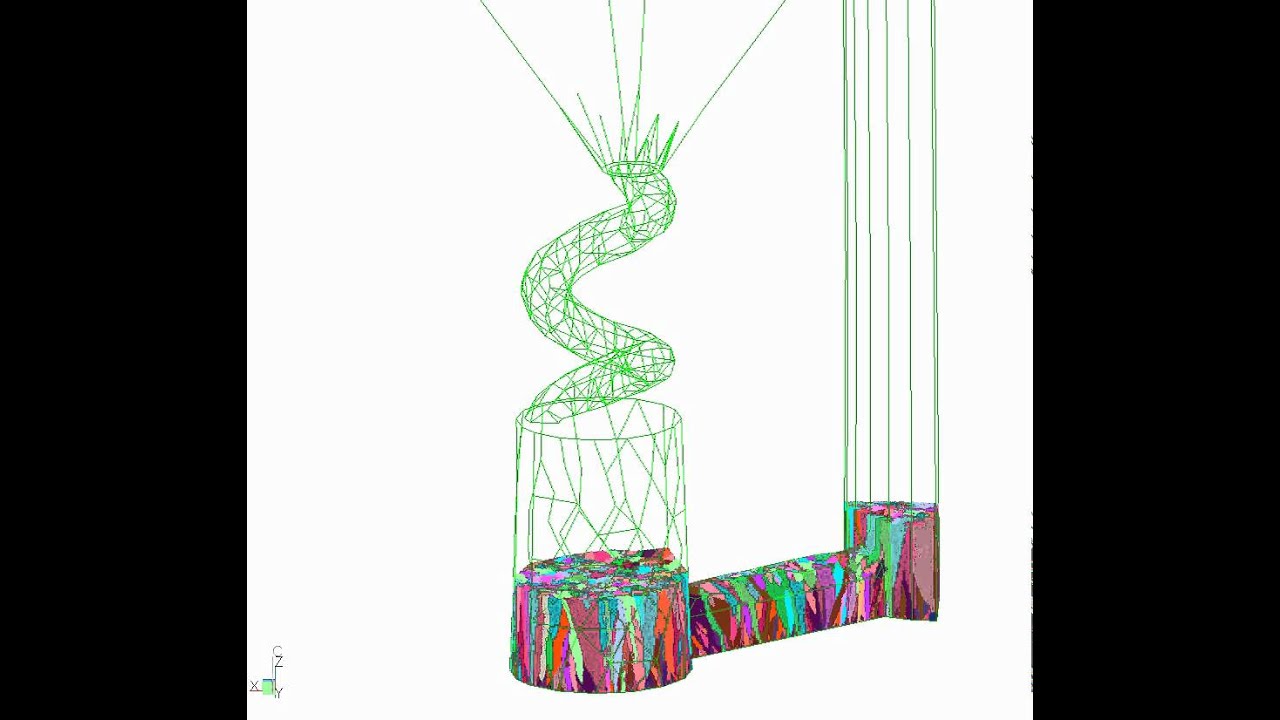
solvent (Figure 4). This method is used to produce large single crystals of Rochelle salt, potassium dihydrophosphate (KDP), naphthalene, and other chemicals.
Single Crystal Xrd
A high-temperature crystallizer (Figure 5) has a crucible with a solvent and the compound to be crystallized. The crucible is placed in a furnace. The compound to be crystallized precipitates from the solvent as the temperature is slowly lowered (solution-melt crystallization). This method is used to produce single crystals of iron-yttrium garnet, mica, and various semiconductor films.
Hydrothermal synthesis of single crystals is based on the dependence of the solubility of the substance in aqueous solutions of acids and alkalies on pressure and temperature. The concentration of the substance in solution and the supersaturation required to produce single crystals are accomplished by means of high pressure (up to 300 meganewtons per sq m [MN/m2], or 3,000 kilograms-force per sq cm [kgf/cm2]) and a temperature difference between the upper part of the autoclave (T1∼ 250°C) and lower part (T2 ∼ 500°C) (Figure 6). The transfer of the substance is accomplished by convective mixing. Hydrothermal synthesis is the main process used to produce quartz single crystals.
The methods used to grow single crystals from a gaseous substance are evaporation of the parent substance in a vacuum, with subsequent precipitation of the vapor onto the crystal, in which case precipitation is sustained by a certain temperature difference T (Figure 7,a); vaporization in a gas (usually an inert gas), where transfer of the substance to be crystallized is accomplished by a directional gas flow (Figure 7,b); and precipitation of the chemical reaction products onto the surface of the seed single crystal (Figure 7,c). The gas-phase crystallization method is used extensively to produce single-crystal films and single crystals for integrated circuits and other purposes.
The selection of the method of growing single crystals is determined by the quality requirements (the number and nature of the defects in the single crystal). A distinction is made between macroscopic defects (extraneous inclusions, blocks, and strains) and microscopic defects (dislocations, impurities, or vacancies).
Special methods exist for reducing the number of defects in a single crystal (such as annealing or growing single crystals on defectless seeds).
Various methods of heating are used in growing single crystals; among them are resistance, high-frequency, gas-flame, and, less frequently, electron-beam, radiation (including laser), and electric-arc heating.
REFERENCES
Buckley, H. Rost kristallov. Moscow, 1954. (Translated from English.)Laudise, R. A., and R. L. Parker. Rost monokristallov. Moscow, 1973. (Translated from English.)
Mullin, J. Kristallizatsiia. Moscow, 1966. (Translated from English.)
Shubnikov, A. V. Obrazovanie kristallov. Moscow-Leningrad, 1947.
Shubnikov, A. V. Kak rastut kristally. Moscow-Leningrad, 1935.
Pfann [W. G.]. “Printsipy zonnoi plavki.” In Germanii: Sb. perevodov. Moscow, 1955. Page 92. (Redkie metally.)
single crystal
Single Crystal X-ray Diffraction
[′siŋ·gəl ′krist·əl] (crystallography)Single Crystal Examples
Want to thank TFD for its existence? Tell a friend about us, add a link to this page, or visit the webmaster's page for free fun content.
Single Crystal Silicon
Single Crystal Turbine Blades
Link to this page:Single Crystal Vs Polycrystalline
